Projects
ACHILLES TENDON REPAIR
We are working with industry partner OrthoCell to develop tools that enable localized Achilles tendon repair using real-time training that targets Achilles tendon strain in a clinical environment.
Musculoskeletal tissues such as tendons are sensitive to their mechanical environment, with both excessive and insufficient loading resulting in reductions of tissue strength. Tendons are particularly sensitive to mechanical strain, with a narrow optimal range of tendon strains that produce positive tendon adaptation.
Our technologies are integrated in a framework to provide real-time feedback of localized Achilles tendon strain during dynamic motor tasks. So far, Achilles tendon localized strains are calculated in real-time during walking, single leg hopping and eccentric heel drop. To do so, we combine 3D tendon imaging, motion capture, wearables sensors, personalised neuromusculoskeletal rigid body models and finite element models.
Our framework connects, across size scales, knowledge from isolated tendons and whole-body biomechanics and offers a new approach to Achilles tendon rehabilitation and training. A key feature is personalisation of model components, such as tendon geometry, material properties, muscle geometry, muscle-tendon paths, moment arms, muscle activation and movement patterns. Due to their inter-individual differences, these components differ substantially between individuals performing the same exercise and potentially affect estimates of their Achilles tendon strain.
Team Leaders: Prof David Lloyd, Prof Rod Barrett, Dr Claudio Pizzolato
Real-time strain of Achilles tendon
Our framework connects knowledge from isolated tendons and whole-body biomechanics and offers a new approach to Achilles tendon rehabilitation and training.
BIOSPINE
Overwhelming scientific evidence has demonstrated how the human nervous system can rewire itself, restoring both lost motor and sensory function in individuals with spinal cord injury (SCI). However, no rehabilitation therapy currently provides the adequate stimuli to the motor and sensory systems to enable rewiring. BioSpine is a novel neural restoration technology that will assemble and control existing, commercially available rehabilitation devices through integration with a Digital Twin of the individual patient.
Our technology has unmatched potential to restore interrupted motor and sensory connections in the spine with the possibility of returning SCI individuals to free movement without assistive devices. BioSpine connects a rehabilitation ergometer, functional electrical stimulation, an electroencephalogram (EEG) reader, and wearable biosensors to create a new class of intelligent rehabilitation devices. A Digital Twin of the patient’s neuromusculoskeletal system and its interaction with the rehabilitation devices is used to create an optimal, personalised sequence of muscle stimulation patterns. This will eliminate the need for a therapist’s manual intervention.
A Digital Twin is used to synthesise the signals from the interrupted spinal connection and redirect them to intact sensory areas via advanced extended reality. The system will initially bridge all the signals from the brain to muscles and back. However, intensive and regular rehabilitation will enable progressive neural restoration and gradual recovery of lost motor and sensory functions in a patient’s lower limbs.
BioSpine also allows the patient to engage in the rehabilitation process by using their thoughts to control the intensity of the rehabilitation program through the EEG reader headset, increasing the potential of improving the quality of life for many people living with SCI.
The BioSpine team comprises researchers from Griffith University, University of Sydney, University of Canterbury and has attracted a key partnership with the world-leading U.S. institutions Spaulding Rehabilitation Hospital and Harvard University. The laboratory on the Gold Coast is equipped with a range of technologies both in use and under development, which will be integrated into a patient-centred design. They include BCI headsets (DSI 7 Wearable Sensing and OpenBCI), Hasomed RehaStim2 FES, system architecture ROS, ergometer, and biosensors with haptic feedback. Our technologies will be designed to retrofit and update existing commercially available equipment.
Team Leaders: Dr Claudio Pizzolato, Dr Dinesh Palipana, Dr Surendran Sabapathy, Dr Chris Carty, Dr Sam Canning, Prof David Lloyd, Prof Rod Barrett, Dr Laura Diamond, Dr Kelly Clanchy, Dr David Saxby, Dr Che Fornusek (UnivSyd), Prof Yang (Ted) D. Teng (Harvard), Dr Nicholas Emerson (UCNZ)
A Digital Twin is used to synthesise the signals from the interrupted spinal connection and redirect them to intact sensory areas via advanced extended reality.
CARDIAC AND VASCULAR PROSTHETICS
Our prosthetics research explores heart valves and artificial hearts. We have a specialisation in the design, development, and testing of cardiac and vascular prostheses including rotary blood pumps (for total artificial heart and ventricular assist applications), blood-filled bearings, heart valves, balloon pumps, and catheters.
We examine the molecular biology, physiology and biophysics of the interactions between mechanical stimuli from fluid flow and biochemical responses in blood cells. In particular, we are exploring various levels of damage to blood cells that has led to new understanding of their survivability to mechanical forces generated by different rates of blood flow.
Furthermore, we have developed a physical test rig, or twin, that mimics the function of the human cardiovascular system and is equipped with our own advanced instrumentation. This system is used to examine blood cell function in different cardiac and vascular prosthetic devices. Armed with this knowledge and test rig we are working with industry to design and test the next generation of heart valves and artificial hearts.
The unique haemodynamic insights we gain (see the project above) allow us to better model and predict how cardiovascular prostheses would perform in both in-silico and physical models. Our modelling capabilities extend to: i. traditional (pulsatile or steady-flow) mock loops for assessing pressure, flow, etc.; ii. blood-filled (pulsatile or steady-flow) mock loops for assessing thrombosis, haemolysis, and other blood damage markers; iii. PIV (particle image velocimetry) for assessing flow patterns (e.g., in pumps, cannulae, and intraventricular); iv. CFD (computational fluid dynamics – Navier Stokes or Lattice Boltzmann method); v. and virtual anatomical fitting and modelling (segmentation of medical tomographs and CAD modelling).
In a current project we are analysing the viability of an intra-ventricular balloon pump for acute and chronic cardiac support using PIV to analyse the flow dynamics at the mitral valve and a blood-filled mock loop to ensure haemocompatibility.
Team leaders: Prof Geoff Tansley, Dr Michael Simmonds, Dr Antony McNamee
Red blood cells and micropipette
We are working with industry to design and test the next generation of heart valves and artificial hearts.
THE DIGITAL ATHLETE
We are developing The Digital Athlete (TDA) from the personalised human digital twin platform, as part of our research into advanced devices for training and rehabilitation in sport.
Instead of guessing whether training, prehabilitation or rehabilitation programs appropriately capture the action and loading of musculoskeletal tissues, TDA uses our capabilities in integrating various personalised neuromusculoskeletal models to offers a non-invasive solution to calculate the action and loading of any musculoskeletal tissues in the body.
This project aims to combine the latest developments in personalised musculoskeletal modelling and multimodal imaging to capture the unique form and function of high-performance athletes. TDA will incorporate internal and external body scans, motion capture and an underlying subject-specific biomechanical model that predicts internal tissue biomechanics in real-time. These data will then be combined to create a high-fidelity model of an individual athlete consistent with animation quality in the big-budget film industry.
The longer-term plan is to work together with relevant partners and industry collaborators to develop TDA for large scale support of elite sport in Australia.
In an unprecedented manner, this technology will identify the fundamental causes of movement deficiencies related to tissues, such as the Achilles tendon and anterior cruciate ligament, and will explore the effect of different interventions on athlete performance. In a significant advance for sports science, TDA can be extended in the longer term to perform computational simulations of athletic performance to answer ‘what if’ questions like “how would greater leg strength affect power output in rowing?”.
The Digital Athlete is a collaborative research endeavour between Griffith University, the Australian Institute of Sport, the Queensland Academy of Sport and Queensland-based industry partners including Myriad Studios and VALD Performance.
Team Leaders: Dr Gavin Lenton (VALD), Dr Matt Bourne, A/Prof Clare Minhan, Dr David Saxby, Prof Rod Barrett, Prof David Lloyd
Bone modelling
Body types
The Digital Athlete will provide coaches and clinicians with a powerful tool to support their decision making.
HAEMODYNAMICS
When blood passes through cardiovascular prostheses, the cells and proteins may be damaged due to the impacts of artificial materials and/or extreme physical forces. Red blood cells may burst, platelets may be activated, and important clotting proteins (e.g., von Willebrand factor) are known to suffer physical destruction.
A particular emphasis of our research is on the subtle effects of shear on blood damage – before a red cell haemolyses, its membrane stiffens, altering its ability to traverse the small capillaries of the microcirculation and indeed altering its dynamics within larger arteries too. Localised ischaemia is suspected to follow such changes and lead to tissue damage.
The Mechanobiology Research Laboratory is uniquely equipped to evaluate these effects bringing together capacity in biorheology, biochemistry, biophysics, engineering and fluid dynamics. We have the capabilities to expose blood to closely titrated doses of shear stress – in bulk or at the individual cell scale – to measure the biophysical, biochemical, and rheological changes and to model these computationally. Our laboratory capabilities include full blood analysis, micromanipulation and visualisation, device design, consultancy and testing, computational modelling and physical modelling. We have a patent pending for a Ventricular Assistance System and Method, PCT/AU/2019/05119.
In a current project we expose single red cells to known amounts of shear and microscopically analyse the differences in trajectories of healthy and damaged red cells in capillary flow. These trajectories are also modelled using Lattice Boltzmann Methods and Immersed Boundary Methods of computational fluid dynamics.
Team Leaders: Dr Michael Simmonds, Dr Antony McNamee, Prof Geoff Tansley, Prof John Fraser
Our research delves into the interactions of blood cells with medical devices and implants
We have the capabilities to expose blood to closely titrated doses of shear stress – in bulk or at the individual cell scale – to measure the biophysical, biochemical, and rheological changes and to model these computationally.
IN-SILICO MEDICINE
In-silico orthopaedic surgeries and implants offer great promise to advance areas of healthcare such as complex orthopaedic surgeries for children. We now designing and creating award winning technology incorporating in-silico surgery and implant design, and 3D printed surgical cutting guides for individual paediatric patients.
For the first time, our personalised digital patient project gave Queensland orthopaedic surgeons the ability to perform surgery on paediatric patients with the assistance of 3D printed cutting guides that have been designed and printed in Queensland for Queensland children. The project provides evidence for the safety and efficacy of simulation informed orthopaedic surgery, with research ranging from digital patient creation, virtual surgery simulation, quality control recommendations, and cleaning and sterilisation validation.
Our team uses the expertise of ADaPT on the Gold Coast campus and works in collaboration with the Queensland Children’s Hospital and two medical device companies, OrthoPediatrics and Surgical Specialties. Together we have recently introduced computer simulations to perform and evaluate surgery in a digital environment before operating on paediatric patients. Most recently, eight surgeries have been successfully performed by surgeons at the Queensland Children’s Hospital. Comparisons to similar surgical procedures performed freehand with traditional surgical planning have demonstrated reduced average radiation dose and time, as well as shorter surgery time.
Our technology will enable clinical facilities to move away from outdated motion analysis methods and place Queensland as both national and international leader in this area of clinical research. It will create local design and manufacture opportunities, medical implementation of custom surgical guides and virtual surgery workflows to facilitate better surgery planning and outcomes for Queensland children with bone deformities.
Team Leaders: A/Prof Chris Carty, Dr Martina Barzan, Dr David Bade, Prof David Lloyd
Osteotomy workflow for in-silico surgeries
Our in-silico technologies will place Queensland as both a national and international leader in this area of clinical orthopaedic research.
SPORTS INJURY PREVENTION
ACL FORCE CALCULATOR
Led by PhD candidate Azadeh Nasseri and in collaboration with colleagues from the University of Melbourne, our team has developed the first validated phenomenological model of ACL loading (ACL force calculator: Patent PCT/AU2019/051129).
The model develops and optimises training methods to prevent and aid recovery from sports injuries related to the anterior cruciate ligament (ACL). We use the computational model to explore differences in ACL forces across maturation in females and examines if and how footwear can change ACL forces. This model will be able to run in real-time for future training applications.
Our research utilises the computing capacity of the School of Health Sciences and Social Work to formulate and test mathematical models of ACL loading.
Team Leaders: A/Prof Chris Vertullo, Dr David Saxby, Prof David Lloyd
Front view of ACL loading
PREVENTING AND MANAGING ACL INJURIES
In collaboration with VALD Performance, we are developing a novel field-test for the assessment of ACL injury risk during dynamic tasks. Once the reliability and validity of the device is established, our team plans to employ it to identify athletes at risk for ACL injury and then to examine the effects of a longitudinal intervention programme targeted at addressing these biomechanical risk factors. In collaboration with Arthrex we are also examining PRP methods of regeneration of Hamstring tendon donor that are used as grafts for ACL reconstructive surgeries. These projects utilise our concurrent technology of The Digital Athlete.
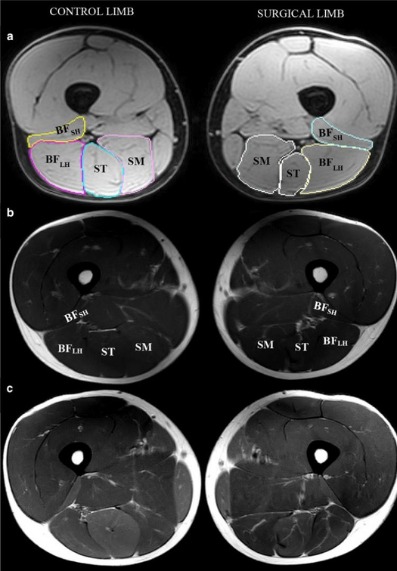
We have developed the first patented phenomenological model of anterior cruciate ligament loading.
HAMSTRING INJURY PREVENTION
We are leading a longitudinal project funded by the Queensland Academy of Sport and supported by Athletics Australia to maximise performance and minimise injury risk in the running athlete, with a spotlight on the hamstrings.
The study aims to explore the impact of targeted training on a) sprint performance and lumbopelvic kinematics; b) hamstring activation and intramuscular coordination; c) knee flexor strength and fatigability; and d) hamstring muscle architecture, aponeurosis geometry, and hip and thigh muscle volumes.
This work will significantly improve our understanding of the effects of different training interventions on sprint performance and known risk factors for hamstring injury. It is expected that it will enable coaches and physiotherapists to make considerably better-informed decisions regarding exercise selection in athletic preparation and injury prevention programmes, leading to fewer injuries and better performances for QAS athletes.
We bring together a multidisciplinary team of researchers from Griffith University and the Queensland University of Technology with expertise in advanced imaging, injury prevention, physiology and biomechanics.
Team Leaders: Dr Matthew Bourne, Dr Steven Duhig, Dr Gavin Lenton, Dr Phil Bellinger
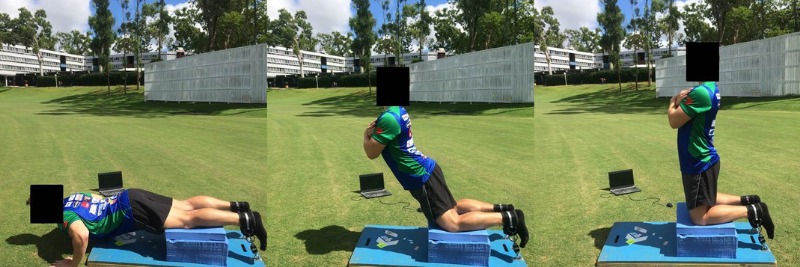
PREVENTING LOWER LIMB INJURIES IN THE FEMALE ATHLETE
In the largest study of its type ever conducted, we are leading a stream of research directed at understanding risk factors for lower limb injury and optimising injury prevention programs for female team sports.
With support from Sports Medicine Australia and VALD Performance, we are currently conducting a large-scale prospective study led by PhD candidate Tyler Collings to explore the association between lower limb strength and performance and subsequent injury in the professional AFL Women's and W-League competitions (involving >350 elite female athletes).
The identification of risk factors for lower limb injuries represents the first step towards designing effective injury prevention and rehabilitation strategies. However, despite a significant amount of research in men’s football there is almost no data relating to risk factors for lower limb injuries in the women’s competitions. Further, there is no data on lower limb strength values for female footballers of different ages, nor is there any data exploring how strength may be altered as a consequence of prior injury. The proposed study aims to fill these gaps and provide team medical and S&C staff with an evidence base from which to develop strategies to mitigate the risk of future injury.
Our research team has travelled around Australia to conduct testing and we utilise Griffiths’ suite of sports science equipment including NordBord, ForceFrame, ForceDecks and HumanTrak.
Team Leaders: Dr Matthew Bourne, Dr Laura Diamond, Prof Rod Barrett
WRIST IMPLANTS
We are developing novel 3D printed and tissue engineered technologies to surgically repair the ruptured scapholunate interosseus ligament in the wrist (the ligament joining the scaphoid and lunate bones, commonly ruptured in falls).
Known as the SLIL Regeneration Project, we are developing a synthetic construct, designed and fabricated by our team, to fit the individual and withstand physiological conditions. The replacement is accompanied by personalised anatomical models for surgeon/patient education as well as customised surgical instruments to aid installation in the body. The replacement is seeded with matured tenocytes using OrthoCell's patented technologies (Ortho-ATI and Cellgro), such that when the construct degrades, new biological tissues take its place. This project demonstrates our capabilities in digital design, computational modelling, medical imaging, robotic testing, advanced 3D printing and regenerative medicine.
The design of these implants is developed by employing personalised digital twins of the wrist and forearm’s musculoskeletal biomechanics, which allows us to test 3D anatomical fit and functional assessment of the final designs. Furthermore, we design the surgical procedures in silico to implant the engineered constructs, and 3D print anatomical models to test the designs and surgical procedures.
We are using a wide range of Griffith University facilities for this project, including ADaPT's digital design and 3D printing, GCORE's robotics and cadaver testing facilities. We are also using University of Queensland's 3D Printing and medical imaging facilities. Further, University of Western Australia's biological testing facilities are used to support the regenerative medicine component of this project.
Our multi-institutional team uses the expertise of the Advanced Design and Prototyping Technologies Institute (ADaPT) on the Gold Coast campus, and represents collaboration with Gold Coast University Hospital, University of Queensland, University of Western Australia and industry partner OrthoCell.
Team Leaders: Prof David Lloyd, Prof Randy Bindra, Dr David Saxby, Dr Laura Diamond, Dr Geoff Tansley, Dr Cedryck Vaquette, Prof Minghao Zheng
Personalised musculoskeletal models drive 3D motion of robotic arm
Preliminary results of the SLIL project show our engineered constructs can withstand physiological loading, are biocompatible, and are viable carriers of stem cells for osteointegration and ligament formation.
Find out more
GCORE research excellence recognised at Vice Chancellor's Awards
Thought control approach to spinal injury rehab raises new hope
Dr Palipana receives global recognition for his passionate advocacy
Innovative Biospine program attracts $2m grant
Beyond spinal cord injury research brings hope on World Science Day
Golesorkhie F, Yang F, Vlacic L and Tansley G, 2020. Field Oriented Control – Based Reduction of the Vibration and Power Consumption of a Blood Pump. Energies, 2020(13):3907, doi: 10.3390/en13153907.
McNamee AP, Tansley GD, Sabapathy S and Simmonds MJ, 2016. Biphasic impairment of erythrocyte deformability in response to repeated, short duration exposures of supraphysiological, subhaemolytic shear stress. Biorheology 53:137-149, doi:10.3233/BIR-15108 .
Pauls JP, Nandakumar D, Horobin J, Prendeville JD, Simmonds M, Fraser JF, Tansley G, Gregory S, 2017. The Effect of Compliant Inflow Cannulae on the Hemocompatibility of Rotary Blood Pump Circuits in an In-Vitro Model. Artificial Organs, 41(10):E118-E128 doi: 10.1111/aor.12919 .
Sahar MSU, Barton M and Tansley GA, 2020. A Systematic Review of the Effectiveness of Cell-Based Therapy in Repairing Peripheral Nerve Gap Defects. Prosthesis 2:153-169, https://doi.org/10.3390/prosthesis2030014.